
One of the oldest medical procedures, palpation, consists of feeling body parts in search of abnormalities that could help diagnosis. For example, a stiffer area on the skin might indicate a tumour mass. But the nature of this physical examination limits information to the most superficial tissues and large abnormalities. What happens if tumours are located deeper inside the body or are too small to be detected manually?
A group of researchers from the Optics and Photonics Research Group at the University of Nottingham may have come up with a solution. In a proof-of-concept study published in Light: Science & Applications, they showed how an ultrasonic probe deployed on the tip of a standard optical fibre can simultaneously determine 3D spatial information and mechanical properties of microscopic structures, generating images with nanoscale depth resolution.
This “phonon probe” could represent the highest resolution ultrasonic 3D fibre imaging device in the world and could pave the way towards minimally invasive point-of-care diagnostics. “We believe its ability to measure the stiffness of a specimen, its bio-compatibility and its endoscopic potential, all while accessing the nanoscale, are what sets it apart,” says Salvatore La Cavera, first author of the study.
Brillouin scattering
Practically, the phonon probe works by pulsing coherent acoustic phonons – synchronized sound particles – into the specimen and detecting the sample’s vibrational response to infer its viscoelasticity.
The probe incorporates two lasers that emit short pulses. One of the laser pulses is absorbed by a gold nanotransducer fabricated onto the tip of the fibre, generating high-frequency phonons that are pumped into the specimen. Then, when a second laser pulse is incident on the specimen, the photons collide with the phonons and undergo a process called Brillouin scattering.
Based on the geometry of this collision, the phonon will either give or receive energy from the photon, resulting in a frequency shift in the light (Brillouin frequency shift). Measuring this shift provides a measure of the sound velocity through the medium. Combining this velocity with the rate at which the specimen dissipates acoustic energy – measured by tracking the decay of the phonon amplitude over time – quantifies the sample’s viscoelasticity.
To demonstrate the probe’s ability to perform parallel 3D topographical mapping and spatially resolved elastography, the team assembled 10-µm-diameter microspheres into complex microstructures and partially melted them to create height irregularities. They used the fibre probe to scan a 100 x 100 µm area centred on the microstructures, in steps of 2 µm.
The probe demonstrated 2.5-µm lateral resolution and could measure height with 45-nm precision, over an order of magnitude smaller than the probe’s optical wavelength.
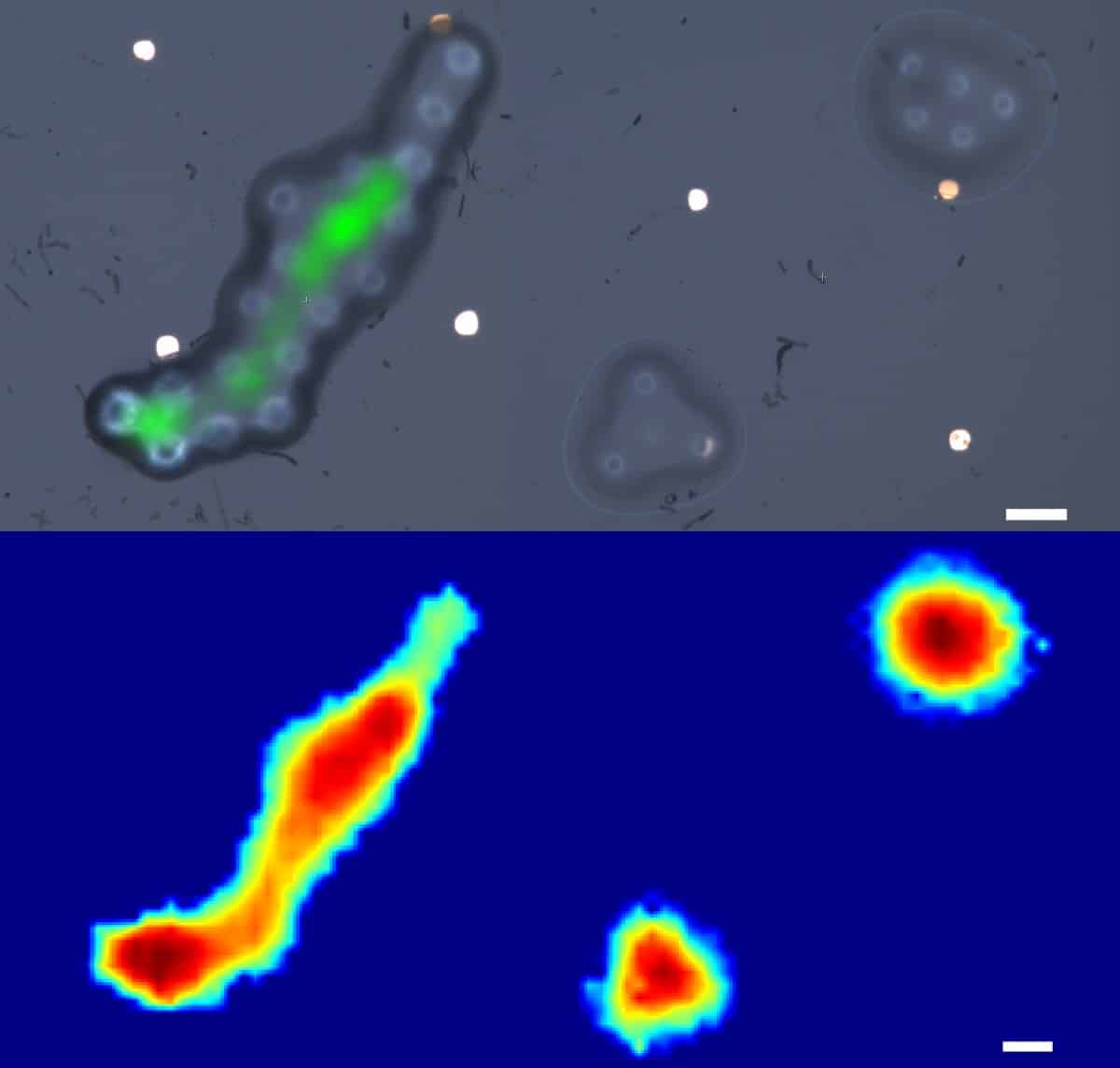
The whole scanning process took 2.8 hr, but the researchers note that in practice, this could be made much faster through sensor optimization. Indeed, probes can be deployed at the tip of each of the 20,000 fibres that make up an imaging bundle, such as in an endoscope, and immediately provide an image of the region-of-interest.
From microscopy to metrology
This is not the first use of Brillouin scattering to assess biomechanical properties of tissues. Brillouin microscopy is already used to characterize tissues in several fields, including cancer biology, tissue regeneration and 3D mapping of corneal biomechanics, where its non-contact and label-free nature make it a method of choice. But the apparatus required is cumbersome, immobile and unsuited to clinical settings, limiting assessments to ex vivo samples.
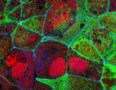
Brillouin scattering reveals tumour dynamics
The phonon probe would provide an immediate inspection tool to help clinicians examine cells within hard-to-reach parts of the body, such as the gastrointestinal tract, and offering more effective diagnoses for diseases ranging from gastric cancer to bacterial meningitis. The team is now looking to develop a series of biological cell and tissue imaging applications to create a viable clinical tool in the coming year.
Looking further afield, the potential of such technology is not limited to healthcare. Fields such as precision manufacturing and metrology could also use this high-resolution tool for surface inspections and material characterization: either as a complementary or replacement measurement for existing scientific instruments.