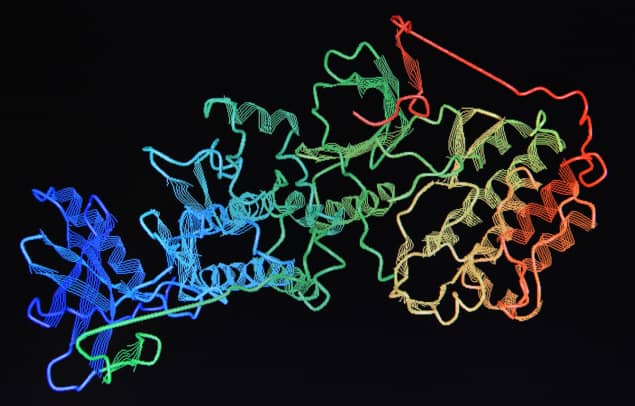
Experimental evidence of long-range attractive forces between cellular proteins has been obtained by researchers in France more than 50 years after the idea was first proposed. The forces are mediated by electromagnetic radiation, and they could explain how molecules find their targets inside the crowded interiors of living cells.
At any given time, around 130,000 pairwise interactions may be occurring between proteins in a living cell. These are mediated by a range of phenomena including van der Waals forces and hydrogen bonding. Biochemical reactions work on a “lock and key” setup, whereby molecules must find and bind to receptors to trigger the processes of life. For this to occur efficiently inside a cell, molecules must find their cognate partners (keys must find the right lock) much more quickly than is possible by simple Brownian motion. However, how this happens so quickly and efficiently is a mystery. One possible explanation is electrostatic attraction, but mobile ions in the cell cytoplasm would screen out static electric fields over ranges longer than 100 nm.
Electrodynamic interactions
In the 1960s, the theoretical physicist Herbert Fröhlich suggested that the resonant exchange of radiation between macromolecules and their cognate partners could be the answer. “Electrostatic interactions are shielded, but electrodynamic interactions are not, in principle, provided these take place at sufficiently high frequency,” explains Marco Pettini of Aix-Marseille University – the work’s principal theorist. Fröhlich reasoned, therefore, that if molecules underwent collective oscillations in an excited state, they might behave like antennas and emit terahertz radiation. If the cognate partner resonantly absorbed this radiation, the two would attract as a result.
Pettini explains, “Biomolecules behave as special kinds of antennas called Hertzian antennas, whose size is much smaller than the wavelength of the radiation, and it’s a consequence of Maxwell’s equations that we can excite these attractive interactions”. However, there was no evidence at the time that proteins could show this collective resonance, so Fröhlich’s suggestion gathered dust.
The principal problem, explains Jérémie Torres of the University of Montpellier, was that the main constituent of a cell’s cytoplasm – water – strongly absorbs terahertz radiation, making terahertz spectrosopy extremely challenging.
Terahertz-frequency fluctuations
In 2018, Torres and colleagues labelled molecules of the protein bovine serum albumin (BSA) with a fluorophore that absorbs 488 nm light. They then dispersed this protein in water and irradiated droplets of the solution with a 488 nm laser. Using a nanowire, they detected terahertz-frequency fluctuations of the electric field around the droplet that matched theoretical predictions from Pettini and colleagues. This showed that proteins could indeed produce collective modes, but not how strong the resulting forces were. Pettini explains, “In principle these forces could be very weak and thus of no interest in biology,” says.
Now in a new paper published in Science Advances, the researchers demonstrate that electrodynamic forces can indeed excite phase transitions in biomolecules. They first use a refined version of their nanowire-based technique to study both the labelled BSA and a second, algal protein that naturally absorbs light. This time, instead of looking simply for collective oscillations in the proteins, the researchers looked at how these oscillations changed when they varied the laser power.
They found that, on increasing the laser power, the frequency of the oscillations shifted, showing that the interaction between the proteins was becoming stronger, as predicted by theory. When they reduced the power, the process was reversed. Moreover, for the algal protein, they used a new, independent spectroscopic technique to show that at high laser powers, the proteins form large clusters: “This is the combination of a very sensitive experimental set-up with a physical mechanism that gives you an absorption that’s even higher than water due to this collective dipole moment,” explains Torres, who led the new experimental work.
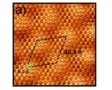
Self-assembled molecular layers strike a balance
The researchers now intend to investigate the effects of these phenomena. Torres points to a 2014 optogenetic study in which two proteins aggregated mysteriously when irradiated by light. “We produce quite similar results but with deeper insight from the physical point of view,” he says. More generally, they want to know how the forces operate in cells, where there are no lasers. “Our work opens a new and broad research topic,” he explains, “During the evolution of life on the planet, nature has exploited all physical phenomena. It would be hard to understand why nature hasn’t resorted to these.”
“It’s a really interesting experiment,” says biophysicist Cécile Fradin of Canada’s McMaster University. “Whether it applies in cell I think remains to be proved and that will determine the ultimate significance, but it’s the first step – well, the second step.” Among several ideas for further work, Fradin suggests that whether proteins and other molecules are resonantly attracted to their cognate partners rather than simply themselves needs investigation. “I would see the next step to be finding out whether you can have two different types of molecules reacting together against a background of all different types of molecules that you don’t want to interact”.