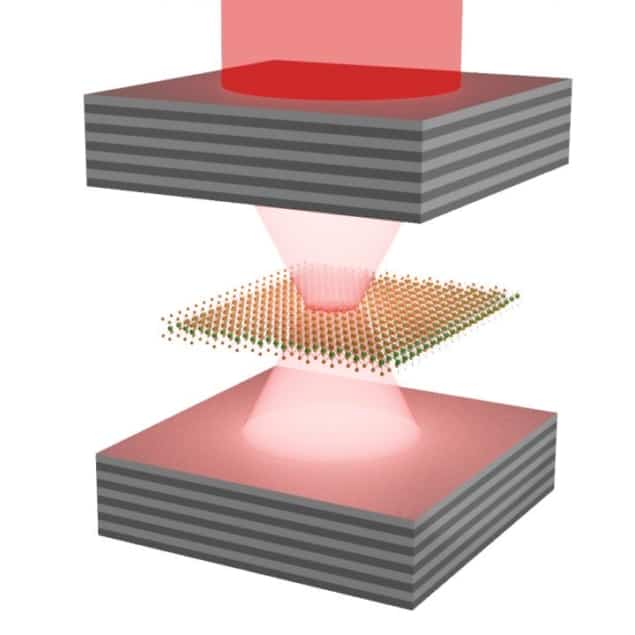
Physicists have taken a step towards realizing the smallest-ever solid-state laser by generating an exotic quantum state known as a Bose-Einstein condensate (BEC) in quasiparticles consisting of both matter and light. Although the effect has so far only been observed at ultracold temperatures in atomically thin crystals of molybdenum diselenide (MoSe2), it might also be produced at room temperature in other materials.
When particles are cooled down to temperatures just above absolute zero, they form a BEC – a state of matter in which all the particles occupy the same quantum state and thus act in unison, like a superfluid. A BEC made up of tens of thousands of particles therefore behaves as if it were just one single giant quantum particle.
A BEC of exciton-polaritons
An international team of researchers led by Carlos Anton-Solanas and Christian Schneider from the University of Oldenburg, Germany; Sven Höfling of the University of Würzburg, Germany; Sefaattin Tongay at Arizona State University, US; and Alexey Kavokin of Westlake University in China, has now generated a BEC from quasiparticles known as exciton-polaritons in atomically thin crystals. These quasiparticles form when excited electrons in solids couple strongly with photons.
“Devices that can control these novel light-matter states hold the promise of a technological leap in comparison with current electronic circuits,” explains Anton-Solanas, who is in the quantum materials group at Oldenburg’s Institute of Physics. “Such optoelectronic circuits, which operate using light instead of electric current, could be better and faster at processing information than today’s processors.”
Anton-Solanas, Schneider and colleagues studied crystals of MoSe2 that were just a single atomic layer thick. MoSe2belongs to a family of materials known as transition-metal dichalcogenides (TMDCs). In their bulk form, these materials act as indirect band-gap semiconductors, but when scaled down to a monolayer thickness, they behave as direct band-gap semiconductors, capable of efficiently absorbing and emitting light.
In their experiments, the researchers assembled sheets of MoSe2 less than a nanometre thick and sandwiched them between alternating layers of silicon dioxide and titanium dioxide (SiO2/TiO2), which reflect light like a mirror. The resulting structure is known as a microcavity and acts like a cage for light. “It’s like trapping the light-emitting material in a room filled with mirrors and mirrors only,” Tongay tells Physics World. “The light gets reflected these mirrors and is absorbed by the material back and forth.”
Sudden increase in light emission
The team cooled the system to 4 K and stimulated it with short pulses of laser light to produce excitons in the MoSe2. These excitons then coupled with light in the microcavity to generate the exciton-polaritons. Using a technique called momentum-resolved micro-photoluminescence spectroscopy, the researchers observed a sudden increase in the light emission from the sample above a certain threshold laser intensity. The researchers say that this, together with the build-up of interference fringes from the polariton light emission, indicates that a BEC had been created from exciton-polaritons.
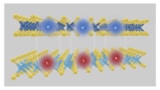
Exciton condensation breaks new temperature record
“In theory, this phenomenon could be used to construct coherent light sources based on just a single layer of atoms,” says Anton-Solanas. “This would mean we had created the smallest possible solid-state laser.”
The researchers, who report their work in Nature Materials, are confident that the effect could also be produced at room temperature in other exitonic materials, such as tungsten-based TMDCs or organic halide-based materials. This means it could be exploited in practical applications. To this end, they are now looking for condensation signatures by studying the interference properties of tungsten diselenide polaritons at room temperature.