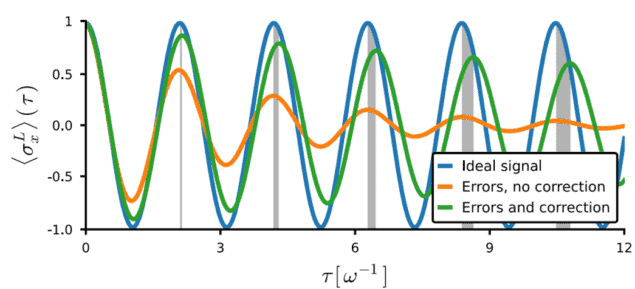
We are used to the idea that in medicine, simple fixes often come with side effects. Taking pain relief for a headache, for example, can leave you drowsy. Now it turns out that a similar principle applies to quantum error correction (QEC). Although QEC protocols are designed to keep quantum devices accurate by eliminating the headache of environmental noise, researchers at ETH Zurich in Switzerland and the Massachusetts Institute of Technology (MIT) in the US have shown that QEC can also introduce bias into the output of quantum sensors. In their analysis, the researchers recommend remedies for this side effect that could prove important in developing commercial quantum sensors for navigation and environmental surveys, among other applications.
Quantum sensors are often better than their classical counterparts at detecting electric or magnetic fields or measuring quantities such as temperature and pressure. However, there is a trade-off: the same quantum effects that give these devices their sensitivity are also very easily destroyed by the equivalent of a crackle in your headphones or glitches on the edges of your computer screen. Because environmental noise can render a quantum sensor’s output inaccurate, QEC protocols are formulated to cancel it out at every step of the sensing process. Each time the sensor performs some action to detect the quantity of interest, the protocol corrects the resulting signal before the next detection occurs – a bit like a sailor correcting a ship’s course as it moves through choppy waters that push it in random directions.
Delayed correction
Crucially, for any realistic quantum sensor, this “steering” cannot happen immediately after the ship starts to veer off. Instead, there is a small delay between the error and the correction, explains Florentin Reiter, a physicist at ETH and the co-author of a study in Physical Review Letters describing the research.
“In the early days of quantum error correction, you simply assumed to have perfect error correction, but that’s just not realistically going to happen. That’s not what we have now,” Reiter says. With sensors based on extremely cold atoms that are controlled by laser light, for instance, Reiter explains that perfect error correction would require those lasers to be infinitely powerful. “QEC is actually being experimentally realized now so we have to be more realistic about it,” he observes.
Quntao Zhuang, a physicist at the University of Arizona, US who was not involved in the study, agrees that we need more realistic and nuanced theoretical models of error correction to fully understand how to make quantum sensors most accurate. “This work explores a problem that was overlooked in the past,” he says. “When you do error correction with operations that have a finite speed, there will be some side effects on how well you can estimate parameters in the system [where sensing is being done].”
Biased measurements
The side effect Reiter and collaborators identified was a consistent bias of the sensor’s output, analogous to a ship tending to drift in the direction of the current. This bias means that all the sensor’s readings will be somewhat inaccurate, and perhaps even unrealistic. What is more, the bias could lead to overly optimistic estimates for the minimum signal the sensor can detect in the first place.
The specific case that Reiter and colleagues considered in their study involved implementing QEC into a procedure for detecting an electromagnetic signal using a system of many identical quantum bits (qubits). However, in the study they demonstrated that their conclusions are true for quantum sensors very generally. Put simply, “when you use error correction, you have to take care of the bias that comes from finite corrections,” says Ivan Rojkov, a PhD student at ETH and the study’s lead author.
The team’s work also contains suggestions for how this side effect of realistic QEC could be remedied. Namely, researchers could anticipate the QEC-induced bias and account for it from the get-go. “If you have full information about how fast you correct and how strong the noise in your system is, you can determine the strength of your error and sort of have a re-calibration of the sensor,” Rojkov explains.
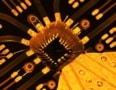
Silicon qubits reach the standard for quantum error correction
While Zhuang points out that implementing error correction in quantum sensors is technically challenging in the near term, and comparable to the task of developing small-scale quantum computing, the ETH-MIT team is optimistic about the impact the work may have in the future. Reiter says that the combination of QEC and bias correction could help physicists push their already extremely precise devices (atomic clocks, for instance, can measure time with an accuracy of 10-15 s) even further. And with several start-ups working to commercialize this technology for applications in navigation, the need for a detailed understanding of quantum effects and quantum information protocols transcends academic research – though Reiter underlines that the new work is relevant for fundamental physics, too. “You could measure tiny relativistic effects and potentially gain better insight into the nature of the universe as given by the most precise values of fundamental constants,” he suggests.