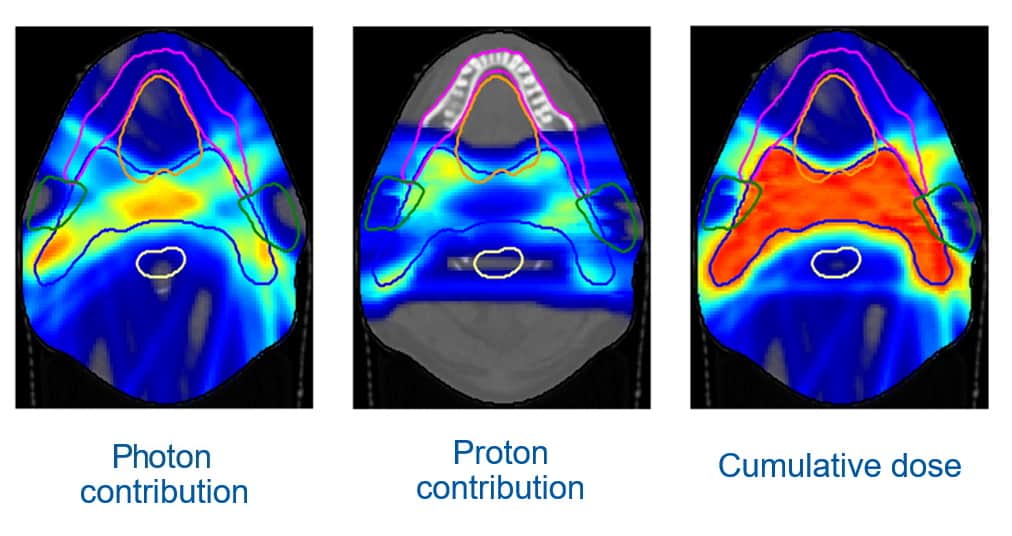
Proton therapy offers precision tumour targeting with low integral dose to normal tissue. But for certain situations, photon-based radiotherapy may hold the advantage. In some cases, the penumbra of a photon beam may be sharper, for example, while proton treatments of lung or breast cancer can suffer from robustness problems.
Proton therapy is also a limited resource, with roughly 100 proton therapy centres worldwide compared with more than 10,000 conventional linear accelerators (linacs). In addition, some facilities only have a fixed proton beamline with limited beam angles, making proton treatments suboptimal. The answer to all of these problems, according to Jan Unkelbach from the University of Zurich, may lie in combined proton–photon radiotherapy. Speaking at the recent AAPM Annual Meeting, he explained the rationale.
The size and expense of proton gantries have hindered the widespread implantation of proton therapy systems. But with the introduction of compact accelerators that can fit into a bunker designed for a standard linac, it becomes possible to install fixed proton beamlines into existing radiotherapy departments more easily and at much lower costs.
“The idea is that we have a treatment room with a robotic couch and a standard linac, and we now add a fixed proton beamline equipped with pencil-beam scanning to that treatment room,” Unkelbach explained. This set-up could deliver both proton and photon beams in the same room, during the same treatment fraction and using the same patient immobilization devices.
Unkelbach described an example of a head-and-neck cancer treatment. With a fixed horizonal proton beamline and the treated patient lying on a couch, the proton beams can only be delivered in the coronal plane, which is not ideal for head-and-neck cancer. “If you can only irradiate from left and right, you have to irradiate through the parotid gland to treat the target volume,” he explained.
Using volumetric modulated arc therapy (VMAT) to deliver photons will better spare the parotid, but delivers a dose bath to surrounding normal tissue. “So we would like to combine protons and photons in an optimal way, and we can do this by simultaneously optimizing intensity-modulated photon and proton plans,” Unkelbach explained. “Indeed, both modalities combined gave the desired dose distribution in the target.”

He presented a similar example for a breast cancer case. Here, the optimal treatment combined tangential photon beams to treat the majority of the target with proton beams to deliver dose to lymph nodes and the target periphery. The team is also evaluating the potential of this approach for many other treatment sites.
Optimizing resources
Photons could also play an important role within existing proton therapy facilities, via combined treatments in which some fractions are delivered using protons and the rest using photons. As well as potentially improving treatment plans (for some patients), the approach could help address limited resources. Ultimately, by distributing proton resources over a larger number of cancer patients it may be possible to increase the benefit of proton therapy for the entire patient population.
In the Netherlands, Unkelbach explained, the decision whether to treat with proton or photon therapy is based on the calculated difference in normal tissue complication probability (NTCP) between a proton plan and a photon plan. “But rather than asking who should receive protons and who should not, could we ask how many proton fractions should each patient receive?” he suggested.
He noted that proton fractions have a diminishing return on the NTCP, with the first proton fraction giving a relatively large benefit, and the last few proton fractions having a smaller impact. There’s also a trade-off between making sure all proton therapy slots are used, while not blocking slots with patients who would only gain a mediocre benefit.
Unkelbach presented the example of a clinic with a single proton therapy system that has capacity for three head-and-neck treatments each day. The clinic treats around 100 head-and-neck cancer patients per year with 30 fractions each, giving an average of 12 patient treatments each day. But with only three proton slots available per day, the goal is to use these proton fractions optimally to minimize the total number of complications seen across all of the patients.
To achieve this, the clinic can perform daily proton slot re-assignment, in which the incremental NTCP reduction is calculated for each patient requiring treatment that day, and proton therapy is delivered to those who would benefit the most from an additional proton fraction. To test this scheme, Unkelbach and colleagues simulated the mean doses to relevant organs-at-risk from intensity-modulated radiotherapy and proton therapy, calculating the NTCP for each.

Protons plus photons: the optimal combination
A simulation of 100 cases resulted in some patients receiving only photons, a few receiving protons only and many receiving combined treatments. Switching all patients from photons to protons reduced the average NTCP by about 15%. But even with only three proton slots available, combined treatments with daily slot re-assignment realised about 5% NTCP reduction – roughly 1% more than the existing patient-based selection scheme.
“Combined proton–photon radiotherapy may enable the development of cost-effective forms of proton therapy , which may allow for more widespread implementation of protons,” Unkelbach concluded. “Secondly, combined treatments with some fractions delivered with protons and others with photons may play a role in optimally using proton resources for the entire population of cancer patients.”