Helsinki University Hospital is working with industry partners to realize the clinical potential of boron neutron capture therapy (BNCT) for complex cancer treatments
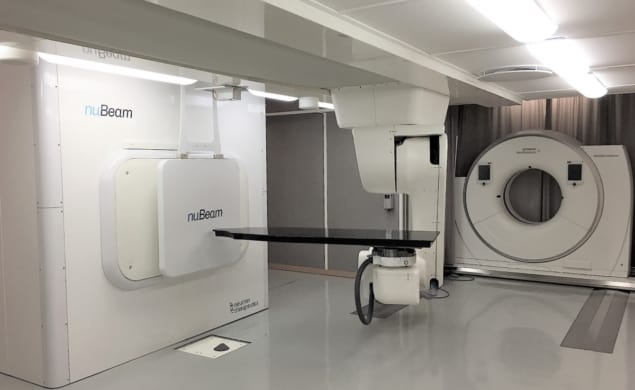
Boron neutron capture therapy (BNCT) is a biologically guided radiotherapy modality that has shown significant promise in clinical trials for the treatment of malignant brain tumours and locally recurrent head-and-neck cancers – complex indications that are difficult to address using conventional radiotherapy techniques. Now, clinicians and physicists at Helsinki University Hospital in Finland – supported by a network of industry partners including Neutron Therapeutics, Siemens Healthineers and BEC – are aiming to take BNCT into the clinical mainstream by exploiting a compact, accelerator-based neutron source that forms part of a purpose-built treatment unit within Helsinki’s Comprehensive Cancer Center.
Commissioning of the accelerator-based BNCT facility is already well advanced, with an initial clinical trial scheduled for mid-2022 on a small cohort of patients (around 30 or so) with inoperable head-and-neck cancers. “The Finnish Radiation and Nuclear Safety Authority has approved the new facility for neutron-beam commissioning,” explains Liisa Porra, project manager of the work-in-progress facility and a medical physicist at Helsinki University Hospital. “Over the coming months,” she adds, “the project team’s focus will shift to verification and validation of the neutron source and the end-to-end treatment workflow, including the patient-positioning robot and in-room CT scanner.”
Biological targeting
In terms of fundamentals, BNCT uses a non-invasive two-step process to target cancer at the cellular level while minimizing collateral damage to surrounding healthy tissue. The first step of the treatment sees patients infused with a tumour-seeking drug (most commonly the boronated amino acid boronophenylalanine) containing a non-radioactive, enriched isotope of boron (10B). Subsequently, the tumour target volume is exposed to a beam of low-energy neutrons, which split the 10B atoms into alpha particles and 7Li nuclei – highly ionizing particles that cause significant damage to the DNA of cancer cells.
Owing to the enhanced accumulation of the 10B carrier in tumour cells, BNCT is characterized by steep dose gradients between cancerous tissue and normal tissue (with significantly more radiation dose deposited in the tumour). “The radiobiology is such that BNCT can be used to treat areas that have been previously irradiated with conventional radiotherapy as well as tumours located adjacent to sensitive organs like the brain stem or the spinal cord,” notes Heikki Joensuu, professor of radiotherapy and oncology at Helsinki University Hospital and senior clinical consultant to the Helsinki BNCT facility.
Joensuu, for his part, is one of the main-movers within Finland’s pioneering BNCT development programme, having worked on clinical trials at the FiR 1 nuclear research reactor in Espoo from 1999 up to its closure in 2012. “We treated more than 200 patients at FiR 1 with malignant brain or head-and-neck cancers,” he explains. “The results demonstrated the clinical efficacy and safety of the technique, while yielding best-practice approaches for BNCT dosimetry and patient/machine QA.”
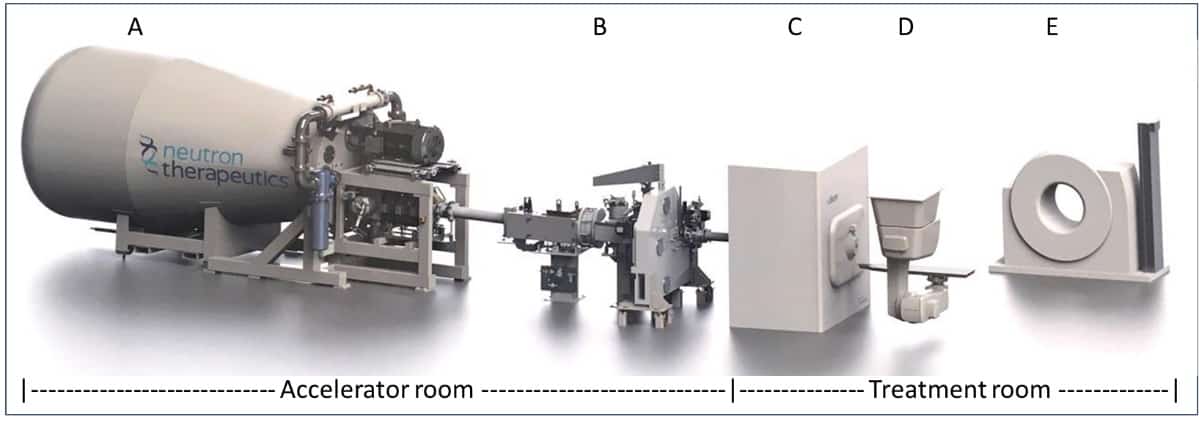
Yet it was the reliance on access to a modified nuclear reactor – a common feature of all BNCT clinical studies over recent decades – that hitherto limited the clinical potential of BNCT R&D efforts. “Our new accelerator-based BNCT facility is located on the hospital campus – a strategic breakthrough that will transform the logistics and economics of patient treatment with neutrons,” Joensuu adds. “Other key innovations – the use of robotic patient positioning and in-room CT for image guidance, for example – will deliver greatly enhanced treatment accuracy, streamlined workflows and increased patient throughput. Having a CT capability in the treatment room will also support future research studies on functional imaging, including the use of functional CT perfusion to evaluate treatment response to BNCT.”
Taking BNCT into the clinic
At the heart of Helsinki’s hospital-based BNCT facility is nuBeam, a compact accelerator-based neutron source from Neutron Therapeutics, a specialist BNCT equipment maker headquartered in Danvers, Massachusetts. The neutron source is housed in a dedicated accelerator and beamline room (65 m2) and comprises a number of discrete building blocks: a single-ended 2.6 MeV electrostatic proton accelerator designed to operate at 30 mA; a beam transport system; an online proton-beam monitoring system; a rotating solid lithium target for neutron generation; a beam shaping assembly (with circular beam delimiter sizes from 8 cm to 20 cm); and an online neutron-beam monitoring system (see figures 1 and 2).
Treatment planning, meanwhile, is based on full Monte Carlo (MC) simulation using CT, MR and PET images, with the planning software defining the patient geometric model including target volumes, organs at risk and their tissue compositions. Worth noting as well that the treatment dose is controlled via direct measurement of the neutron fluence by the online beam-monitoring detectors (rather than relying on calibrated proton current data).
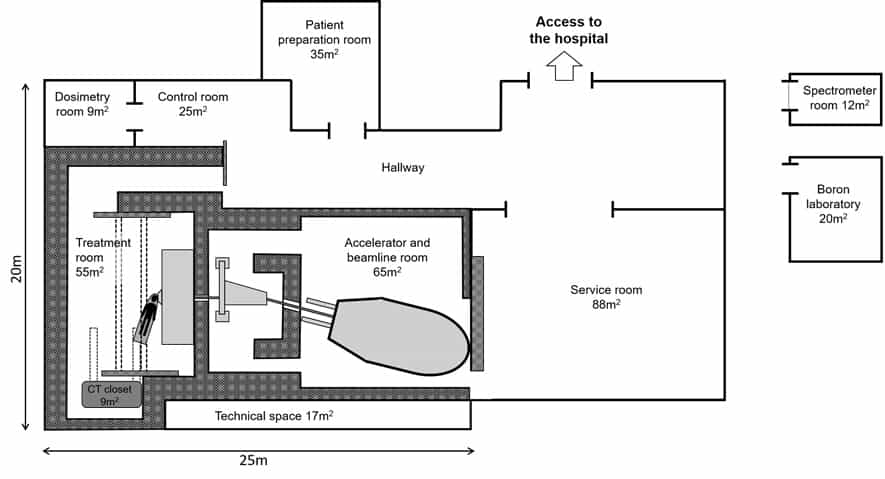
Downstream from the nuBeam source, the patient treatment room (55 m2) is fitted out with a robotic patient positioning system, exacure, from German medical technology specialist BEC. The custom-modified industrial robot can not only be positioned in all three dimensions, and with six degrees of freedom, but also be moved along the ceiling towards and away from the neutron-beam nozzle. An integrated optical tracking system monitors the treatment couch position 500x per second to apply corrections (accurate to ±0.5 mm) for patient positioning and CT imaging. The latter is performed with a rail-mounted Siemens Healthineers SOMATOM CT Sliding Gantry, which takes in-room CT images for comparison with the planning images to ensure precise patient set-up ahead of treatment delivery.
“Patient positioning for BNCT is even more challenging than for conventional radiotherapy modalities,” explains Mikko Tenhunen, chief medical physicist at Helsinki University Hospital. “The horizontal nature of the neutron beam means we will often require two or even three unique couch set-ups to deliver a range of treatment fields.”
More broadly, implementing a new BNCT workflow from scratch brings its own unique set of challenges. Any equipment placed near the neutron beam – the positioning robot, for example – is covered with neutron-absorbing material to inhibit activation, while all patient-support devices – such as pillows, cushions and restraints – are tested for susceptibility to neutron activation before being cleared for use in the treatment room. The treatment facility is also equipped with a high-purity Ge gamma spectrometer for neutron activation analysis; paired ionization chambers with water and PMMA phantoms for estimating neutron and gamma-ray absorbed dose to reference tissue; and an inductively coupled plasma optical emission spectrometry device for analysis of blood-boron concentration prior to treatment.
Due diligence
Right now, Tenhunen and colleagues are laser-focused on equipment commissioning in advance of the BNCT clinical trial next summer. “All of the main subsystems of the BNCT facility have been tested separately,” he says, “while end-to-end validation of the treatment workflow will follow later this year. Ultimately, clinical success is all about how the various subsystems integrate together to deliver optimum outcomes across treatment planning, treatment delivery and treatment management.”
The outlook, it seems, is bright for the clinical application of BNCT technology. “The new Helsinki facility will be the first in-hospital BNCT treatment system in Europe,” concludes Joensuu. “It’s a significant landmark – one that we hope will promote clinical validation and wide-scale adoption of BNCT by cancer treatment centres around the world.”
- Liisa Porra will present a progress report on the Helsinki BNCT programme at the Biology-Guided Adaptive Radiotherapy (BiGART 2021) virtual symposium on 6 October.